Forfattere: Diana Lindberg1, Runar Gjerp Solstad1, Jan Arne Arnesen1, Anita Kaupang Helmers1 & Ragnhild Dragøy Whitaker1
1 Nofima AS – Norwegian Institute of Food, Fisheries and Aquaculture Research, Muninbakken 9-13, NO-9019 Tromsø, Norway (www.nofima.no)
Referanse: Lindberg, D., R.G. Solstad, Arnesen, J.A., Helmers, A.K. & Whitaker, R.D. (2021). Økonomisk fiskeriforskning, 31: 1-2021, pp. 42-57.
Abstract
An evaluation of value-creation starting from snow crab (Chionoecetes opilio) side streams into various products, utilizing different processing regimes, has been performed. A maximum recovery of 43.7% protein and 37.4 g/kg oil were achieved using proteolysis on shells with entrails. From empty shells, 23.8 mg/kg recovery of carotenoids was achieved, and approximately 100 g/kg of chitin was recovered. The developed processes were evaluated for scalability and avenues for industrial production in order to evaluate commercial opportunities, as well as illustrate the use of demonstration plants in the commercialization phase.
Sammendrag
Ved bruk av forskjellige prosesseringsmåter ble en evaluering av verdiøkningspotensialet av snøkrabbe (Chionoecetes opilio) sidestrømmer utført. Flere produkter er blitt produsert. Basert på hydrolyse av skall med innvoller ble et maksimalt utbytte på 43,7 % protein og 37,4 g/kg fett fra råstoffet oppnådd. Studiet viser videre at prosessering av skall muliggjør en utvinning av 23,8 mg/kg av karotenoider og cirka 100 g/kg kitin. For å vurdere kommersielt potensiale er prosessene som er utviklet blitt evaluert for oppskaleringspotensiale og måter for industriell produksjon. I tillegg ble muligheter for å benytte eksisterende demonstreringsanlegg i kommersialiseringsfasen vurdert.
Content
Introduction
Snow crabs are an abundant and commercially important species in Bering Sea, East Canada, and West Greenland (Lorentzen, 2018). Snow crab fisheries have been rapidly increasing in Norway since 2013. The landings in Norway increased rapidly with a peak in 2016 with a total landing of about 11 000 tons before these numbers sharply declined in 2017 (SSB Norway, 2020). The decline was due to most of the Barents Sea loop hole being closed for Norwegian fishing vessels, and the national quota was set at 4000 tons (Nærings- og fiskeridepartementet, 2019) In 2019, the quota was again set to 4000 tons while in 2020, the quota was increased to 4500 tons (Hjelset, 2019).
Many investments have been made in order to catch, process, and introduce the Norwegian snow crab to the domestic and international market (Lorentzen, 2018). To make the fisheries as profitable as possible, it is important to maximize the value of all the biomass that is caught. When processing snow crabs into the conventional product, i.e. processed clusters (Lorentzen, 2020), much of the biomass can be lost as waste. This biomass is the body of the crab, which is often discarded when the crab is taken on board at sea, after the legs and claws are separated from the body, cooked and then frozen. As the fisheries is conducted far from shore, the crab is commonly processed directly on the fishing vessel, as keeping the crabs alive and in good quality back to land for processing is complicated due to snow crab behaviour and environmental requirements (Lian, 2018; Siikavuopio, 2019). If the catches are done closer to shore, the crabs can be brought live to shore or exported live to selected markets (Lorentzen, 2018). The main reasons this waste biomass is discarded is that there are no established processes to collect and handle the bodies, and processes designed to recover high value products from the entire biomass are not established. In addition, the markets for products derived from co-products from snow crab are not established (Lorentzen, 2018).
From a commercial perspective, the component groups of interest in crustacean shell co-products are proteins (including enzymes), carotenoids, lipids, and chitin. Various studies have been published aiming at investigating extraction and characterization of these components from snow crab co- and by-products. These studies has been aimed at characterising these components extracted from both soft and hard snow crab carapace, without hepatopancreas and haemolymph (Shahidi & Synowiecki, 1991), snow crab carapace with hepatopancreas and haemolymph (Beaulieu, 2009), and snow crab shells only (Asunción Lage-Yusty, 2011). Studies has also been aimed at investigating amino acid and protein quality of snow crabs and its co-products (Vilasoa-Martínez, 2007), characterization and extraction of proteolytic enzymes (Souchet & Laplante, 2011), and bioactive peptides extracted from the snow crab co-products (Beaulieu, 2010; Alain Doyen, 2011; A. Doyen, 2012; El Menif, 2019). In general, two kinds of biomasses have hence been investigated, carapaces with or without intestines. The most industrially accessible coproduct is the carapaces without intestines. This is because in the industrial process of removing the clusters, the remainder crab carapace is separated from the clusters in a process using excess water such that the content is commonly washed out, resulting in an available biomass mostly consisting of shells with little contents. One can argue that this co-product the most relevant for chitin and carotenoid extraction as the relative concentration of chitin and carotenoids, being bound in the carapace structure, would be higher in a co-product consisting of crab carapace only.
Several protocols for extraction of astaxanthin involves the use of toxic solvents (Honda, 2019), such as acetone, a highly flammable organic solvent. However, in the Shahidi & Synowiecki study, an interesting extraction protocol based on use of the then inexpensive cod oil was utilized, and a concomitant maximum 74% yield in extraction of carotenoids was achieved (Shahidi & Synowiecki, 1991). This could provide a more sustainable process from the viewpoint of removing the need for use of highly flammable organic solvents. Since then, the price for cod oil has increased, but evaluation of more inexpensive oils could be of interest as a sustainable alternative in carotenoid extraction.
Extraction protocols for chitin extraction from crustacean shells involves at least a demineralisation and a deproteinisation step, which can be used in any which order, and many times also a bleaching step. It is preferable to perform the extraction in a way as to utilize the other components in the shells at the same time (Synowiecki & Al-Khateeb, 2003). A deproteinisation aimed at extracting valuable proteins before chitin extraction performed by use of strong alkaline solutions, as performed by Shahidi & Synowiecki (Shahidi & Synowiecki, 1991), can lead to racemization of L-amino acids to undesired D-amino acids and partly destroys amino acids such as arginine, tyrosine, lysine, cysteine and threonine (Kristinsson & Rasco, 2000). Beaulieu and co-workers investigated the chitin yield after hydrolysis, but rather as a starting point for chitin extraction than performing a full extraction including demineralisation and deproteinisation.
On the other hand, the use a co-product based on carapace with both haemolymph and hepatopancreas intact allows for extraction of both a protein and an oil fraction, as well as the use of the hepatopancreas endogenous proteases for hydrolysis (Eisen, 1973; Klimova, 1990; Souchet & Laplante, 2011). Indeed, Beaulieu and co-workers performed enzymatic protein hydrolysis with a commercial protease in pilot scale to extract and characterize the protein, ash, oil, and mineral fraction starting from filled carapace (Beaulieu, 2009). However, the activity of the endogenous proteases in this process were not investigated. This could be interesting from a commercial perspective, as commercial proteases often are considered as one of the important economic variables in enzymatic protein hydrolysis-based processes (Aspevik, 2017).
In this context, the aim of this study was to evaluate if use of the two classes of snow crab biomass now considered as waste can be utilised in production of valuable products for one or more plausible markets. Several processes aimed at recovery of single components has been performed. The protocols were aimed at either improving, provide a more sustainable alternative to, and/or reduce the cost of processes as described in earlier publications. Focus was placed on using green chemistry and simple processes, meaning that harsh solvent and solvent that require explosion proofing in processing plants were avoided. These restrictions were made to enable the products to be cleaner and facilitate implementation in existing production plants, including the demonstration plant Biotep. Based on yield, process time required to perform these processes to isolate the different products, calculations aiming at estimations on processing costs in a demonstration phase through use of an accessible demonstration plant, Biotep, has been performed. Lastly, evaluations on if the products could be processed in large scale and if there could be a market for such products has been conducted.
Materials & Methods
The experimental animal
In September 2016, male snow crabs were harvested using snow crab pots in the Barents Sea and transported live to the Aquaculture Research Station in Tromsø, Norway and immediately placed in 6 m3 tanks of seawater. After a week, the crabs were transported in a dry state, in polystyrene boxes covered with gel ice from the Research Station to Nofima in Tromsø, live stored at 4 ˚C over night, and slaughtered the day after.
Co-products
For the protein and oil extraction from full snow crab carapaces, including haemolymph and hepatopancreas, the average snow crab size was approximately 740 g. Co-products from 41 animals were comminuted in a UM12 grinder (Stephan Machinery GmbH, Hameln, Germany), aliquoted and flushed with N2, whereafter the single packages were frozen at -20 ˚C until the day of experiment.
For carotenoid extraction and the trial combining protein hydrolysis and chitin extraction, snow crabs were caught in the Barents Sea in October 2018 and transported on land. Crab shell without viscera were collected directly after slaughter, frozen at -20 ˚C, transported to Tromsø and stored at the same temperature until further use. Approximately 45 kg of frozen crab shells were thawed overnight and comminuted in a meat mincer (Kilia). Crushed shells were further homogenized in a Waring blender. After grinding, the shells were aliquoted into smaller packages and stored at -20 ˚C until the day of experiment.
Protein and oil extraction by enzymatic protein hydrolysis
In both protein and oil as well as chitin extraction, hydrolysis was performed in a Symphony 7100 system (Distek, North Brunswick, NJ). In the protein and oil extraction, 450 g of ground snow crab co-products from full shells were mixed with an equal amount of water. After reaching a temperature of 50 ˚C, 0.5% (w/w) of protease was added. The following proteases were used: Endocut 01 (Tailorzyme, Herlev, Denmark), and Protamex (Novozymes, Bagsvær, Denmark). After running the hydrolysis for 120 min, the reaction mixture was heated to 90 ˚C for protease inactivation and the reaction mixture was then kept at this temperature for 10 min. In the next step, the insoluble shell fractions were separated from the liquid phase with a commercial sieve. The liquid was further centrifuged (Jouan KR4i, VWR, Norway) at 7000 x g for 20 min, resulting in three fractions: water, fat, and sediment. The water phase was stored frozen at -20 ˚C for further analysis.
The snow crab material for enzymatic protein hydrolysis was analysed for water using an internal method at TosLab (Tromsø, Norway), Kjeldahl protein content according to NMKL 6 (NMKL, 2003) and ash content using ISO 5984:2002. The full snow crab co-product was analysed for amino acid composition based on the method ISO 13903:2005, as stated by Commission Regulation EC 152/2009.
Carotenoid extraction
Four different oils with different fatty acid composition, namely soybean, palm, olive, and cod liver were used for carotenoid extraction. Olive oil, palm oil and soybean oil were purchased from Sigma Aldrich whereas cod liver oil was bought at a local pharmacy. The differences in fatty acid composition of saturated, mono-unsaturated fatty acids (MUFA) and poly-unsaturated fatty acids (PUFA) are found in Table 1 (source Matvaretabellen.no, Norwegian food safety Authority, 2020).
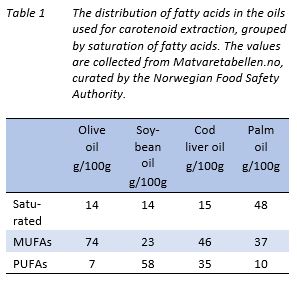
The extraction was performed in the Symphony 7100 system described in “Protein and oil extraction”. Two different carotenoid extraction procedures were tried: use of 1:1 oil:carapace (w/w), and use of three extractions with 1:3 oil:carapace (w/w), all rounds with a duration of 30 min, at 60 ˚C. Since three extractions meant a higher level of energy applied (3 × 30 min), the former extraction procedure (1:1) was performed once with the same amount of time spent under heating as the latter experiment (1:3), but without removing and adding oil between experiments. Reference value extractions were carried out using acetone instead of oil with dried carapace, using the dried equivalent of the wet weight of carapace, at the same ratios, as for oil extractions.
The method used for establishing the amount of free astaxanthin and astaxanthin esters in snow crab shells, oils, and acetone extracts are the in-house validated methods A23 and A101, respectively, performed at the accredited commercial analysis lab Biolab (Bergen, Norway).
Chitin extraction from shells after enzymatic protein hydrolysis
The same material used for astaxanthin extraction was also used for chitin extraction. The enzymatic protein hydrolysis was performed using the same equipment and methodology as described in ”Protein and oil extraction by enzymatic protein hydrolysis”. However, the amount of water and snow crab shells used in this subtrial were 400 g (w/w), the temperature during hydrolysis was 60 ˚C using a stirring rate of 100 rpm. 0.75% (w/w) Alcalase 2.4 L was added at t = 0, and after 30 min, the same amount of Flavourzyme was added. Both enzymes were produced at Novozymes (Bagsværd, Denmark). The reaction was run for 60 min, before being terminated using the same protocol as earlier described. After inactivation, a separation of hydrolysate and remaining crab shells was performed. The crab shell remains were washed using 200 ml water and stored at 2 ˚C until chitin extraction.
Washed shells from two identical enzymatic protein hydrolysis treatment were pooled for isolation of chitin. The isolation of chitin was performed in two steps, either by first performing a deproteinization, or first performing a demineralization. Deproteinization was performed as described earlier (Gildberg & Stenberg, 2001), but with small changes to shorten the process. The protocols are presented in Table 2. 180 g shell was used in each treatment. For each step during the protocols, solids were separated from solvents by a sieve.
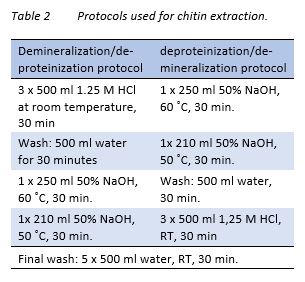
The chitin yield was calculated by the equation: (w dry chitin/w shells) x 100%. Ash content was determined by gravimetry on the remains of a determined mass after heating at 540 ˚C for 24 hours.
Estimation of production costs for the various components if produced at Biotep
Nofima owns and operate a demonstration plant, Biotep. This plant has been operational since 2014 and has been used to process a large variety of biomasses, including various shellfish and biomass from snow crab.
The demonstration plant can be rented on an hourly basis, and commonly productions are performed renting the plant for one week. In the calculations, established prices for Biotep and the expected throughput in this demonstration plant together with the yield obtained on a laboratory scale were used to estimate how much it would cost to produce one kilo of the different isolated products. The expected throughput from productions at Biotep are not published in scientific reports, but the cost structure and throughput estimates are available on the web (Biotep, 2020). At Biotep, the plant is rented at 2800 NOK per hour, in addition, the producing entity covers the variable costs, including steam and electricity which is the largest expenditure of variable costs. An average work week with continuous production is 104 hours including rigging of the production and cleaning post-production (Biotep, 2020). Steam and electricity usage depend on the temperature employed during processing, as well as how much drying is required. Some products are dried to a powder while other products are dried to a semi-dry product (Vega-Mercado, 2001). The dried powder takes longer time and requires more energy to produce but is commonly cheaper to transport and store than the semi-dried compounds. Some products like oils are kept and shipped in liquid form. The format of the finished product will depend on the further use and must be evaluated for each product (Petrova, 2018).
Based on the capacity at Biotep, the estimated throughput was 500 Kg per hour. This amount is based on an average of comparable productions and is the throughput that is used when estimating production costs. These estimates are performed when a production contract is signed with Biotep (Biotep, 2020). Other production plants will have different throughput capacity and cost structures, and this will affect the production cost (Frishammar, 2015; Hellsmark, 2016). The estimated throughput for Biotep was used in these investigations because it enables the usage of established rental costs at Biotep, having the right infrastructure in place to process the biomass. Based on empirical numbers at Biotep, out of one work week (104 hours) 6 hours are estimated with no productivity for preparation and cleaning, which results in a theoretical maximal throughput of 49 tons per week.
Experience shows that throughput per week varies between 20 to 46 tons, however, in the processes described here, the estimates at the low end is set to 30 tons biomass in per week and at the high end to be the maximal throughput of 46 tons per week (personal communication with production team at Biotep). The variable costs for products that are evaporated or dried are set to 60 000 NOK per week, and to be about 20 000 NOK per week with no drying and little heating (personal communication with production team at Biotep). Thus, the total price for one week of production is 104*2800 NOK = 291 200 NOK per week, plus variable costs. The cost per kilo product is then dependent on biomass throughput on one week (between 30 and 46 tons) and the output of product, for example the percent output of proteins. This forms the basis for the kilo prices given in the results section.
Results and discussion
Protein and oil recovery from full snow crab shells
A trial to investigate use of endogenous and three exogenous proteases for hydrolysis on snow crab shells containing haemolymph and hepatopancreas was performed.
Raw material characteristics
The amount of protein, fat, and ash in the raw material, given in g/100 g of both wet and dry weight is presented in Table 3. Earlier protein values reported on co-products from snow crabs as determined by Kjeldahl method, i.e. %Nitrogen x 6.25, are 17.79% (dry weight) after KOH hydrolysis on empty soft shell snow crab carapace (Shahidi & Synowiecki, 1991), 33.0% on empty shells (Vilasoa-Martínez, 2007) and 42.9% (dry weight) in filled snow crab shells (Beaulieu, 2009). The latter raw material mostly resembles the co-product in this study, although the fat content of the snow crab shells was reported to be 14.8% instead of the current co-product containing 25% of fat on a dry weight basis. As noted in the Beaulieu article, the actual proximate distribution will vary with small changes within the biomass from experiment to experiment.
The hydrolysis products
The percentage of protein and ash of the hydrolysates are given in Table 4. As seen, there were no differences in protein amount in the hydrolysates, all reaching values over 60%. However, the ash content of the dried hydrolysates differed more, with the hydrolysates resulting from endogenous proteases resulting in the lowest levels. Endocut-01 hydrolysis resulted in the highest overall ash content, and a relatively low protein yield. Earlier work on the protease resulting in the highest yield, Protamex, on hydrolysis of snow crab shells reported on hydrolysates containing 57.7% proteins, 20.8% ash, and 11.1% lipids (Beaulieu, 2009). It is reasonable to expect the lipid content of the hydrolysates in the present study to be approximately the same as the sum of protein and ash contents were equal to that seen in the Beulieu study (Beaulieu, 2009).
As seen in Table 4, results suggest that the best overall protein recovery was seen after Protamex digestion, resulting in a 47% protein recovery from the proteins in the start material. After hydrolysis, the sediment and oil fraction were separated from the water-phase and the weight recovery of the oil was noted. The isolated protein and oil fraction recovery given in g/kg of snow crab co-products after hydrolysis and oil separation are given in Figure 2.
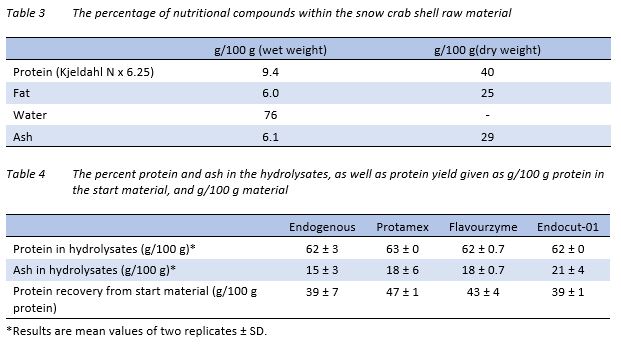
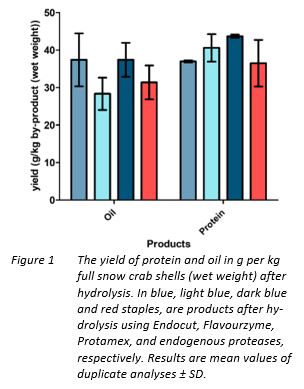
Although the best protein recovery seem to result from the Protamex reaction, the large errors resulting from hydrolysis using only endogenous proteases, or use of Flavourzyme suggest that for being able to conclude on the best protease, further trials need to be conducted. In the work by Beulieu (Beaulieu, 2009), the protein recovery resulting from hydrolysis of full snow crab shells using 0.1% Protamex was 51%, in a hydrolysis reaction were a temperature of 40 ˚C was used. The protein recovery after the current study was lower, 47%, using five times the Protamex concentration (0.5% (w/w)) at a temperature of 50 ˚C. One explanation for the difference in protein recovery could be the fact that there was raw material variation between the different trials. Beaulieu reports on 42.9 ± 4% proteins and the presence of small amounts of left-over meat (Beaulieu, 2009) , while the protein content of the current study material was 40% based on a material sans left-over meat. Protamex is stated to have an optimum temperature at 60 ˚C, and based on use of both a higher concentration and running the reaction at a temperature closer to the optimum temperature in the current study, the higher yield resulting from the Beulieu study is still surprising. One additional explanation might be the contribution of the endogenous proteases. From the current study, it is clear that the endogenous proteases contribute significantly to the protein recovery. Proteases from hepatopancreas of cold-adapted crabs such as snow crab but also red king crab, could be expected to have an optimum temperature closer to the 40 ˚C used in the Beaulieu study. As an example, a study by Semanova and co-workers (Semenova, 2008) on red king crab hepatopancreatic proteases, showed that at one isozyme of the king crab serine proteases had an optimum temperature range between 38 ˚C–40 ˚C. If that is true also for snow crab proteases remains to be investigated, but if verified, implies that process optimization by use of endogenous only might result in acceptable protein recoveries without the addition of oftentimes costly commercial proteases.
Carotenoid extraction
In the present investigation, snow crab carapace without fill was prepared for carotenoid extraction, as these showed it to contain the highest relative amounts of carotenoids of all parts of the snow crab co-products (Shahidi & Synowiecki, 1991). Further, the extraction was performed without pre-treatment to avoids decomposition of carotenoids (Shahidi & Synowiecki, 1991). One well-recognized method to extract astaxanthin and its derivates in the carotenoid family is by acetone extraction (Gimeno, 2007), and this was used as the standard method. In this study, both the more expensive cod liver oil and three different and inexpensive plant oils with varying fatty acid profiles (Table 1) were used, namely olive, palm, and soybean oil. Two alternative extraction protocols were investigated, aiming at comparing if a 1:1 ratio (oil:shell) extraction at 60 ˚C, 30 min, was equally effective as a 3 times 1:3 ratio (oil:shell) extraction using the same temperature and time. The latter 1:3 ratio protocol involved the use of comparatively more energy than the 1:1 ratio protocol, with the crab shells being warmed three times to 60 ˚C. Therefore, a test where a 1:1 ratio of olive oil:shell was heated three times to 60 ˚C, 30 min, with a cooling period in between was performed. The results are presented in Figure 3, A-B.
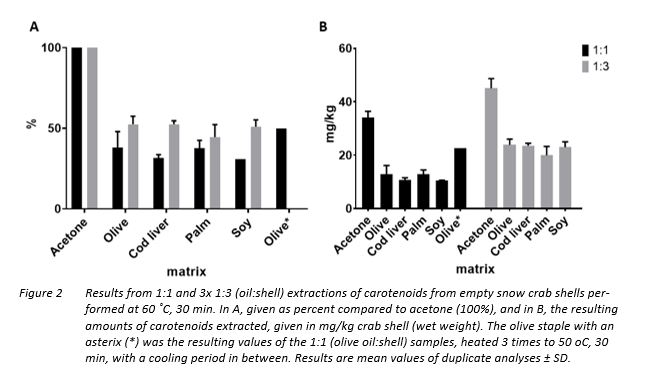
Using two methods of analysis, one giving the amount of free astaxanthin, and one resulting in the sum of astaxanthin esters, it was established that the amount free astaxanthin in all cases were less than 10% of the sum of astaxanthin esters extracted from the crab shells. Among astaxanthin esters, the sum of diesters were in all cases higher than the sum of monoesters.
The maximum sum of astaxanthin esters extracted in the current study was achieved using the reference acetone method, yielding a recovery of 45 mg/kg snow crab co-product using the 1:3 protocol. When comparing the extraction yield in mg/kg resulting from the two protocols, the 1:3 extraction protocol clearly resulted in higher overall yields for both oils and acetone. There was no clearly preferred oil for extraction based on the current study results, however, results indicate that the palm oil was least efficient and olive oil most efficient. Interestingly, the 3 x 1:1 protocol investigated on the olive oil, marked with an asterix in Figure 3 (A and B), resulted in approximately the same yield as the 1:3 protocol. This indicates that a more easily facilitated heating of oil:crab shells in 3x 1:1 extraction might be as efficient as the 1:3 extraction protocol.
This study resulted in a relatively lower sum of free astaxanthin relative to the total amount of carotenoids extracted, as compared to the extracted amounts of free astaxanthin in the Shahidi & Synowiecki study, namely 21% in hard shell and 31% in soft shell. The lower values seen in the current study could be a result of astaxanthin degradation due to oxidative reactions during extraction and freezing, or due to reactions with oxidative species within plant and marine oils themselves. The same distribution of a relatively higher sum of diesters to monoesters, however, was also observed in the mentioned study (Shahidi & Synowiecki, 1991).
The obtainable yield of carotenoids is likely affected by the amount of carotenoids in the shells, in turn dependent on feed and season (Daly, 2013). This makes absolute comparisons harder, but higher yields of carotenoids than achieved in the current study are likely to be obtained by process-related factors, such as use of more oil, higher temperature, and longer mixing time (Sachindra & Mahendrakar, 2005). Use of a 2:1 protocol (oil:snow crab shell co-product) at 60 ˚C resulted in 74% carotenoid extraction (Shahidi & Synowiecki, 1991). When it comes to finding the most suitable oil, several studies have been conducted aiming at finding the most suitable oil for astaxanthin extraction of carotenoids from other crustaceans than snow crab, and many have concluded that vegetable oils are preferable to marine oils. A study including different marine oils (herring, menhaden, salmon) and vegetable oils for recovery of carotenoids from crawfish by-products concluded that soybean oil resulted in the highest carotenoid recovery (Chen & Meyers, 1984). However, the plant oil resulting in the highest yield vary. Studies on extraction of carotenoids from shrimp by-products using seven different plant oils, including soybean oil, shows that it is sunflower oil that yields the highest recovery of 26.3 mg/kg shrimp by-product using a 2:1 ratio of oil/by-product (Sachindra & Mahendrakar, 2005). The current results indicate no clear preference in choice of oil, as both cod liver as well as soybean and olive oil resulted in almost the same yields. Clearly, as seen from the other studies mentioned that also performed a screening of several oils for carotenoid extraction, the factors contributing to the most successful oil in extraction are complex and warrant further investigations. On the other hand, having the choice of several oils with approximately the same yield allows for product optimisation for different markets interested in different oils. Also, when it comes to process optimisation and the use of for example higher oil-to-by-product ratios, longer extraction time, and higher temperatures, this will come at the expense of concomitantly higher production costs.
Chitin/chitosan extraction
Chitin extraction was performed on the solid phase of snow crab shells after enzymatic protein hydrolysis using a combination of Al-calase and Flavourzyme. After protein hydrolysis, 67.5% (dry weight) of the shell fraction remained. The remains were pooled and subjected to chitin extraction. To investigate if the order of demineralisation and deproteinisation mattered in respect of yield and ash content, an important quality parameter, extraction using either of these two options was performed. The processing is likely to deacetylate parts of the chitin into chitosan, but no attempts has been made to investigate the distribution of the two components in the product. The yield of dried chitin/chitosan product extracted from crab shells was 10.5% of the total wet weight for demineralisation first, and 10.6% for deproteinisation first. This correspond to ca 100 g chitin-derived product extracted per kg snow crab shell. The ash content was 0.87% for demineralisation first, and 0.11% for deproteinisation first. Based on the dry weight of the shells, the weight yield of the chitin-derived product was 25.1% or 26.9% for demineralisation or deproteinisation first, respectively.
Earlier studies have reported on different amounts of chitin in the snow crab shell material. Working with full snow crab shells, Beaulieu and co-workers (Beaulieu, 2009) reported a chitin content of 16% of the total dry weight. Shahidi & Synowiecki determined chitin content in empty snow crab shells, concomitantly reporting on a higher percentage of 26.6% in the dried shell material (Shahidi & Synowiecki, 1991). Based on the latter amount, also based on use of empty shells, the results from the current study indicates that most of the chitin from the snow crab shells were extracted using either protocol. However, it is important to note that the chitin percentage in crustacean shells vary over the year (Lian, 2021; Rødde, 2008), which means that the current recovery estimate from the start chitin amount could be overestimated.
There are numerous protocols published on how to extract chitin from crustaceans (Arbia, 2013; Hamed, 2016; Synowiecki & Al-Khateeb, 2003). The purpose of the current study was to use a simple method for extraction of a crude product, rendering the basis for recovery estimates which can be used for estimations on the economics in production at an existing processing plant. It was also important to utilise a process enabling extraction of valuables from a previous step, in this case protein hydrolysis. An estimated recovery of close to 100%, based on the Shahidi & Synowiecki chitin content in snow crab shells indicates both methods performing adequately. However, the higher ash amount of the protocol involving demineralisation first suggests that a protocol involving a deproteinisation first is preferable. To be able to draw further conclusions on product quality and hence market possibilities however, deeper studies on product characteristics linked to processing e.g. degree of deacetylation, molecular weight, as well as for example colour must be performed (Arbia, 2013). To investigate the feasibility of extraction of chitin, protein, and astaxanthin from snow crab shells in a stepwise process, further and deeper studies are underway.
Estimation of processing costs and the use of demonstration plants in an early commercialisation phase
Many articles indicate the potential usage of crab shell for value creation (Beaulieu, 2009; Su, 2019; Tremblay, 2020), however in order to proceed in this work there is a need to evaluate whether the products can be made at scale in a cost effective way, and whether there is any market interest, it would be valuable to test production and estimate some production costs for industry in Norway. To evaluate the processing costs of the side streams from snow crab estimates, previous processes performed at Biotep have been used, described in materials and methods. The cost structure for Biotep is based on a non-profit operation of the demonstration plant. At Biotep, similarly to many demonstration plants, the infrastructure is flexible and thus designed to be adaptable to many processes. This is beneficial in the testing of processes as it allows for scaling of many different productions in a demonstration phase, however, it also leads to a larger biomass loss than in processing plants that are designed to run very specific processes (Frishammar, 2015; Hellsmark, 2016; Nordqvist & Frishammar, 2019; Whitaker, 2021).
However, using a demonstration plant will allow the producers to test the process, and evaluate the kind of infrastructure that should be used in the processing of various biomasses, and it allows for testing of a prototype of a product in the market. The prototype testing in the market allows for both evaluating market entry as well as getting B2B and B2C feedback in order to optimize the product. This kind of prototype testing in the market will allow for investigation of the type of customers that can be targeted, labelling or communication around the product, as well as the willingness to pay for the customers in the targeted market.
Initial investigations of proteins and isolation of proteins in crab shells including the intestines were performed. While this is not the form the shells are commonly collected at the plants, the contents of proteins and oils from the empty shells are very low, and in order to create value from these constituents, the shells with contents was evaluated to be more likely to have a commercial potential. Collecting the contents would typically require a form of sieve or grating and containers that could collect intestines in the slaughter process. The possibilities for value creation from collecting and processing the shells and side streams must be evaluated together with the possible marketable products. Protein products from snow crab can for example be introduced as a healthy ingredient in products or as a marine protein nutraceutical. The largest addition to the market from marine proteinaceous sources in later years are marine collagen preparations from marine sources, but marine proteins that are not pure collagen has also attracted interest in many fields, including sports nutrition (Mjøs, 2019). One specific example of this in Norway, is a product made from salmon hydrolysate (Framroze, 2016) which is produced by both Hofseth Biocare and Biomega in Norway. Marine proteins can be used towards both food and feed applications, with the nutraceuticals and cosmeceutical markets being markets with high paying customers (Pleym, 2019; Svorken & Pleym, 2019). Another segment in the high end of the market are bioactive compounds, where the market is in rapid growth (Coherent market insight, 2020; Daliri, 2018). For bioactive peptide products, a demonstrated bioactivity that is approved by a regulatory body, which in the EU would be recommended EFSA and approved by the commission would likely attract a much higher price. However, the developmental costs and market entry strategy carries a significant cost and work efforts (Altintzoglou, 2021; Calado, 2018; Whitaker, 2021). Bioactive claims are relevant for functional food, functional beverages, nutraceuticals, animal nutrition and personal care products. For all functional protein products, the market drivers has been indicated, including an increased awareness of nutrition in an aging population and a population with increased health focus (Coherent market insight, 2020). In addition, an increased awareness for sustainable food sources and reduction of food waste can be seen among consumers (Altintzoglou, 2021), Thus products from biomass that is commonly wasted can be attractive based on being sustainable. The sales prices for marine proteins vary greatly based on product, but in personal communication with industry and food producers, the authors have experienced that a bulk protein product sold as an ingredient to human consumption is more likely to net between 100-300 NOK per kilo depending on product. If the protein is used as ingredient for petfood, the price is likely between 25 and 40 NOK per kilo. Therefore these are indicative numbers, and the price obtained from a marine product is likely to be better estimated if a prototype product can be presented to producers and producers are allowed to evaluate possibilities for the protein to be incorporated in a specific product. This is possible when using a demonstration plant, and thus the Biotep estimates have been included in this study.
The extraction of protein has not been performed in industrial scale, but we used the cost estimates described in Material and Methods which is based on the experience from operation of the Biotep plant, average production throughput at this plant, established production costs at the this plant, and knowledge that the demonstration plant has the appropriate infrastructure to scale and produce the different products (Biotep, 2020). With the production capacity described in material and methods, the price per kilo at Biotep is estimated to be between 167 NOK per kilo and 255 NOK per kilo. As mention above, these are only production costs and do not include other parts of the supply chain, including collection, transportation, further refining and sales/distribution of the product (Hobbs, 2002). When planning a production, these estimates indicate that the margins can be small if the production were to be run at that volume in a demonstration plant on a regular basis. A thorough evaluation of possible market entry and sales channels must be made before embarking on production. Ideally a test production at scale will give valuable input to the commercial potential, then the producer needs to evaluate how much the process can be optimized, if other products can be included, and how much production costs can be reduced or if sales income can be increased. The role of a demonstration plant is to test the technology and the product in a market, and help the industry move past the demonstration phase (Fletcher & Bourne, 2012; Frishammar, 2015; Hellsmark, 2016; Nordqvist & Frishammar, 2019).
Production costs can be reduced by optimizing the process, but also through isolating more than one product in the same production. With regards to process optimization, this can be achieved by reducing time, temperature and/or increasing yield. It can be seen from Beaulieu et al that a shorter hydrolysis time at lower temperature than used in the current study (40 ˚C versus 50 ˚C) with Protamex resulted in a higher yield (Beaulieu, 2009). As discussed above this can possibly be attributed to an increasing activity of the endogenous enzymes at the lower temperature, as Protamex is expected to have very low activity at that temperature. One challenge with autolysis (breakdown using endogenous enzymes) is that it is a less controlled process as the activity and levels of endogenous enzyme vary with feeding status, weight, age etc of the snow crab (Hardy, 2000).
Another way of increasing the value of the side streams is by isolating more than one product during the process. It can be seen from the results that in addition to proteins, the hydrolysis process results in oil from the crab intestines and a small portion from the shells. This oil fraction is generated at the same time as the protein product and is thus coisolated. By also valorising this fraction, the commercial potential of the production is increased. A large variety of omega 3 arctic marine products are available on the market, commonly sold in 250-500 mL bottles (Marketsandmarkets, 2019) with a large price range. From Norwegian websites an average of about 1000 NOK per litre was observed. With a density of about 0.9 kg per litre, the price per Kg oil is estimated at 1100 NOK. The composition of marine omega-3 oils on the market is similar to oil from snow crab, with just under 20% of the oil being EPA and DHA fatty acids in the oil (Beaulieu, 2009). By accounting for the oil yield using the hydrolysis process, an estimated 36 Kg oil can be expected from each ton of processed wet biomass. Using the same estimated processing amounts as for the proteins, the cost per Kg of oil is estimated to be between 200 and 315 NOK per litre at Biotep. It is expected that this oil will need to be processed further in order to remove any pollutants and heavy metals, however with the expected sales prices, there is room for value creation from collection of the proteinaceous and the oil phase from processing of snow crab side streams in the same production. Thus, from a hydrolysis process and a one week of production, the result can be a protein and oil prototype product that can be tested in the market. As the fisheries is expected to increase in the next several years, the production volume of side stream can also increase, and the costs can go down. But in an initial phase, the costs at the demonstration plant Biotep and possible market prices can be an attractive commercial avenue (Frishammar, 2015; Hellsmark, 2016; Nordqvist & Frishammar, 2019; Whitaker, 2021).
Another possible value creation that has been evaluated is using empty shells, where the contents are rinsed off in the slaughter process as described earlier, which is the way they commonly arise in processing facilities. Another already mentioned possibility is to use the shell left over after hydrolysis, where the protein contents are hydrolysed away as described, which makes it suitable for chitin and astaxanthin isolation from these biomasses.
Chitin was extracted from the crab side streams as described above. Chitin has a wide variety of applications in the market, from ingredients in food and feed stuffs, to cosmetics, medical devices, biodegradable and antibacterial packaging and agricultural causes (Heide, 2020). The prices vary greatly depending on molecular mass, degree of acetylation, crystalline structure, colour and purity (Arbia, 2013). Deep characterization of these properties will assist in determining a more likely market price, however that is beyond the scope of the initial investigations of commercial application of the side streams. The current study results show that about 10% of the total mass of the crab shells can be recovered. Prices for chitin products range from 140–400 NOK per Kg for the very low range to 1200 NOK per gram (Heide, 2020; Arbia, 2013). Using the throughput estimates at Biotep as described above, the cost per kilo of production chitin at Biotep is between 300 and 1000 NOK per kilo, highly varying depending on how much shells are being put through in one week of production and the time consumed. The processes are initial and cost estimates are only indicative. If crab shells should be used for chitin production, a thorough characterization of the product as well as commitment from the market for a verified volume above the total costs including production, logistics and sales. However, with a high throughput, there is a potential for value creation from the chitin. The large differences between low and high throughput is due to the large percent composition of chitin in the biomass.
In addition to isolation of chitin, various oils were employed to extract carotenoids from the shells. The highest amount of astaxanthin that was isolated was about 25 mg/kg. The astaxanthin is dissolved in the oil, thus this can be a product being sold as it is directly, as an enriched oil. Shiahidi & Synowiecki suggested that fish oils with carotenoid pigments to have the added advantage of offering feeds rich in omega-3 fatty acids (Shahidi & Synowiecki, 1991). It can also be used it as any oil with antioxidants to feed (Chen & Meyers, 1982). With the latter, however, in order to get the real value from the astaxanthin, further processing and clean-up will be required, thus a processing price is difficult to estimate at the Biotep. The carotenoids can also be used in high end marine oil with sustainable marine antioxidants, replacing synthetic antioxidants (Olatunde & Benjakul, 2020). The prices for astaxanthin range significantly depending on purity from as high as 6000 NOK per gram to about 1000 NOK per Kilo (Heide, 2020). From a week of production at Biotep, the extraction of on average 25 mg astaxanthin per kilo crab shell has the potential to yield over a kilo of pure astaxanthin, with the cost per gram being about 280 NOK per gram on the high end of throughput as can be expected in this type of method where no drying is applied. Astaxanthin has been more successfully extracted from crustacean side streams using supercritical carbon dioxide (Ahmadkelayeh & Hawboldt, 2020). As described below, this can be a more relevant and sustainable technique in the future.
Conclusion
The aim of this study was to evaluate whether the biomass that is now waste from the snow crab industries can be used for production of valuable products in one or more plausible markets. The evaluation was based on use of protocols aimed at recovery of single components. Focus was placed on using green chemistry and simple processes, implementable at the small industrial scale demonstration plant, Biotep. The practical production in demonstration scale was estimated using empirical numbers from previous productions at Biotep of comparable biomasses and the pricing structure at Biotep. The authors concluded that proteins and oil from intact crab housing could be extracted in scalable processes with processing costs that could result in a profitable product. In addition, astaxanthin and chitin could be isolated from empty crab shells, however the processes needs optimization before being scaled. Processes should be well demonstrated in smaller scale before the cost of demonstration production is invested in. In addition, the prototype resulting from a demonstration production should be well tested in market.
Further work
Although optimisation work on extraction of protein, oil, carotenoids, and chitin could result in higher yields and lower production costs, and in the case of proteins, more refined products, the highest potential could lay in being able to extract more than one component at the time.
In addition, as mentioned, supercritical carbon dioxide has been identified as a more sustainable future technique for lipid soluble components, including carotenoids. These techniques will be further evaluated in new equipment being installed in accessible laboratories and is intended to be installed for larger scale productions.
In addition to the process development, other important elements also need to be investigated to get a good basis for the commercial use of side streams from snow crab. To mention a few, these include knowledge about competitors activities, the status and activities in other snow crab fishing nations, identification of competitors in the endmarket and their activities, and how these ingredients can meet the needs and demands of the endcustomer/user. In addition, in production of new products, one must identify how the candidate products can be differentiated from similar ingredients already present in the market.
Acknowledgements
Financial support from the Research Council of Norway “SnowMap” (no. 267763), through RFFNORD “SnøRest” (no. 248229), and internal financing from Nofima through the project “Peptek” is greatly acknowledged.
References
Ahmadkelayeh, S., & K. Hawboldt (2020). Extraction of lipids and astaxanthin from crustacean by-products: A review on supercritical CO2 extraction. Trends in Food Science & Technology, 103, pp. 94–108.
Altintzoglou, T., P. Honkanen & R.D. Whitaker (2021). Influence of the involvement in food waste reduction on attitudes towards sustainable products containing seafood by-products. In review In N. AS (Ed.). Journal of cleaner production. (Accepted 2020-12-08).
Arbia, W., L. Arbia, L. Adour & A. Amrane (2013). Chitin Extraction from Crustacean Shells Using Biological Methods – A Review. Food Technology & Biotechnology, 51:1, pp. 12–25.
Aspevik, T., Å. Oterhals, S.B. Rønning, T. Altintzoglou, S.G. Wubshet, A. Gildberg, N.K. Afseth, R.D. Whitaker & D. Lindberg (2017). Valorization of Proteins from Co- and By-Products from the Fish and Meat Industry. Topics in Current Chemistry, 375:3, p. 53.
Asunción Lage-Yusty, M., M. Vilasoa-Martínez, S. Álvarez-Pérez & J. López-Hernández (2011). Chemical composition of snow crab shells (Chionoecetes opilio) Composición química del caparazón del cangrejo de las nieves (Chionoecetes opilio). CyTA – Journal of Food, 9:4, pp. 265–270.
Beaulieu, L., J. Thibodeau, P. Bryl & M.E. Carbonneau (2009). Characterization of enzymatic hydrolyzed snow crab (Chionoecetes opilio) by-product fractions: a source of high-valued biomolecules. Bioresour Technol, 100: 13, pp. 3332–3342.
Beaulieu, L., J. Thibodeau, M. Desbiens, R. Saint-Louis, C. Zatylny-Gaudin & S. Thibault (2010). Evidence of Antibacterial Activities in Peptide Fractions Originating from Snow Crab (Chionoecetes opilio) By-Products. Probiotics Antimicrob Proteins, 2:3, pp. 197–209.
Coherent market insight (2020). BIOACTIVE PROTEIN & PEPTIDES MARKET Global Industry Insights, Trends, Outlook, and Opportunity Analysis, 2020–2027. Available at: https://www.coherentmarketinsights.com (Accessed: 5 December 2020).
Biotep (2020). www.biotep.no
Calado, R., M. Costa Leal, H. Gaspar, S. Santos, A. Marques, M.L. Nunes & H. Vieira (2018). How to Succeed in Marketing Marine Natural Products for Nutraceutical, Pharmaceutical and Cosmeceutical Markets. Cham, Switzerland: Springer
Chen, H.-M. & S. Meyers (1984). A rapid quantitative determination of astaxanthin pigment concentrate in oil extraction. JAOCS, Journal of the American Oil Chemists’ Society, 61, pp. 1045–1047.
Chen, H.-M. & S.P. Meyers (1982). Extraction of Astaxanthin Plgment from Crawfish Waste Using a Soy Oil Process. Journal of Food Science, 47:3, pp. 892–896.
Daliri, E.B.-M., B.H. Lee & D.H. Oh (2018). Current trends and perspectives of bioactive peptides. Critical Reviews in Food Science and Nutrition, 58:13, pp. 2273–2284.
Daly, B., J.S. Swingle & G.L. Eckert (2013). Dietary astaxanthin supplementation for hatchery-cultured red king crab, Paralithodes camtschaticus, juveniles. Aquaculture Nutrition, 19:3, pp. 312–320.
Doyen, A., L. Beaulieu, L. Saucier, Y. Pouliot & L. Bazinet (2011). Demonstration of in vitro anticancer properties of peptide fractions from a snow crab by-products hydrolysate after separation by electrodialysis with ultra-filtration membranes. Separation and Purification Technology, 78:3, pp. 321–329.
Doyen, A., L. Saucier, L., Beaulieu, Y. Pouliot & L. Bazinet (2012). Electroseparation of an antibacterial peptide fraction from snow crab by-products hydrolysate by electrodialysis with ultrafiltration membranes. Food Chem, 132:3, pp. 1177–1184.
Eisen, A.Z., K.O. Henderson, J.J. Jeffrey & R.A. Bradshaw (1973). Collagenolytic protease from the hepatopancreas of the fiddler crab, Uca pugilator. Purification and properties. Biochemistry, 12:9, pp. 1814–1822.
El Menif, E., C. Offret, S. Labrie & L. Beaulieu (2019). Identification of Peptides Implicated in Antibacterial Activity of Snow Crab Hepatopancreas Hydrolysates by a Bioassay-Guided Fractionation Approach Combined with Mass Spectrometry. Probiotics and Antimicrobial Proteins, 11:3, pp. 1023–1033.
Pleym, I.E., M. Svorken & B. Vang (2019). Verdifulle rester. Muligheter for norsk marint restråstoff. Report 9/2019, Nofima, Tromsø, p. 26).
Fletcher, A.C., & P.E. Bourne (2012). Ten Simple Rules To Commercialize Scientific Research. PLOS Computational Biology, 8:9, e1002712.
Framroze, B., S. Vekariya & D. Swaroop (2016). A Placebo-Controlled, Randomized Study on the Impact of Dietary Salmon Protein Hydrolysate Supplementation on Body Mass Index in Overweight Human Subjects. Journal of Obesity & Weight Loss Therapy, 06.
Frishammar, J., P. Söderholm, K. Bäckström, H. Hellsmark & H. Ylinenpää (2015). The role of pilot and demonstration plants in technological development: synthesis and directions for future research. Technology Analysis & Strategic Management, 27:1, pp. 1–18.
Gildberg, A. & E. Stenberg (2001). A new process for advanced utilisation of shrimp waste. Process Biochemistry, 36:8, pp. 809–812.
Gimeno, M., J.Y. Ramírez-Hernández, C. Mártinez-Ibarra, N. Pacheco, R. García-Arrazola, E. Bárzana & K. Shirai (2007). One-Solvent Extraction of Astaxanthin from Lactic Acid Fermented Shrimp Wastes. Journal of Agricultural and Food Chemistry, 55:25, pp. 10345–10350.
Hamed, I., F. Ozogul & J. Regenstein (2016). Industrial applications of crustacean by-products (chitin, chitosan, and chitooligosaccharides): A review. Trends in Food Science & Technology, 48, pp. 40–50.
Hardy, D., J.-D. Dutil, G. Godbout & J. Munro (2000). Survival and condition of hard shell male adult snow crabs (Chionoecetes opilio) during fasting at different temperatures. Aquaculture, 189:3, pp. 259–275.
Heide, M., T. Altintzoglou & R.D. Whitaker (2020). The market for marine ingredients for food and agricultural Application – Coldwater prawn (Pandalus boralis), Blue mussels (Mytilus edulis) and Brown crab (Cancer pagurus). Report 32/2020, Nofima, Tromsø, p. 9.
Hellsmark, H., J. Frishammar, P. Söderholm & H. Ylinenpää (2016). The role of pilot and demonstration plants in technology development and innovation policy. Research Policy, 45:9, pp. 1743–1761.
Hjelset, A.M., C. Hvingel, H.E.H. Danielsen, J.H. Sundet, O.-B. Humborstad, T. Jørgensen & S. Løkkeborg (2019). Snøkrabbe på norsk sokkel i Barentshavet – Bestandsvurdering og kvoterådgivning 2020. Available at: (Accessed: 5 December 2020) https://www.hi.no/resources/Snokrabbe-pa-norsk-sokkel-i-Barentshavet-Bestandsvurdering-og-kvoteradgivning-2020.pdf (Accessed: 5 December 2020) In Havforskningsinstituttet (Ed.).
Hobbs, J.E. (2002). Evolving Supply Chains in the Nutraceuticals and Functional Foods Industry. Canadian Journal of Agricultural Economics/Revue canadienne d’agroeconomie, 50:4, pp. 559–568.
Honda, M., H. Kageyama, T. Hibino, Y. Zhang, W. Diono, H. Kanda, R. Yamaguchi, R. Takemura T. Fukaya & M. Goto (2019). Improved Carotenoid Processing with Sustainable Solvents Utilizing Z-Isomerization-Induced Alteration in Physicochemical Properties: A Review and Future Directions. Molecules, 24:11.
Klimova, O.A., S.I. Borukhov, N.I. Solovyeva, T.O. Balaevskaya & A. Strongin (1990). The isolation and properties of collagenolytic proteases from crab hepatopancreas. Biochemical and biophysical research communications, 166:3, pp. 1411–1420.
Lian, F., I. Måge, G. Lorentzen, S.I. Siikavuopio, K. Øverbø, B. Vang & D. Lindberg (2018). Exploring the effect of inhibitors, cooking and freezing on melanosis in snow crab (Chionoecetes opilio) clusters. Food Control, 92, pp. 255–266.
Lian, F., S.I. Siikavuopio, A. Hustad, T. Thesslund, S.-K. Lindberg & G. Lorentzen (2021). Comparative quality evaluation of processed clusters obtained from red king crab (Paralithodes camtschaticus) typical of spring and autumn harvests in the Barents Sea. In. Submitted to Food Control.
Lorentzen, G., F. Lian & S.I. Siikavuopio (2020). Live holding of snow crab (Chionoecetes opilio) at 1 and 5 °C without feeding — Quality of processed clusters. Food Control, 114, 107221.
Lorentzen, G., G. Voldnes, R.D. Whitaker, I. Kvalvik, B. Vang, R.G. Solstad, M.R. Thomassen & S.I. Siikavuopio (2018). Current Status of the Red King Crab (Paralithodes camtchaticus) and Snow Crab (Chionoecetes opilio) Industries in Norway. Reviews in Fisheries Science & Aquaculture, 26:1, pp. 42–54.
Marketsandmarkets. (2019). Omega-3 Market by Type (DHA, EPA, and ALA), Application (Dietary Supplements, Functional Foods & Beverages, Pharmaceuticals, Infant Formula, and Pet Food & Feed), Source (Marine and Plant), and Region – Global Forecasts to 2025.
Mjøs, I., E. Thorsen, T. Hausken, E. Lied, R.M. Nilsen, I. Brønstad, E. Edvardsen & B. Frisk (2019). The effect of low dose marine protein hydrolysates on shortterm recovery after high intensity performance cycling: a double-blinded crossover study. Journal of the International Society of Sports Nutrition, 16:1, p. 48.
NMKL. (2003). Nitrogen, Determination of foods and feed according to Kjeldahl. NMKL method no 6, 4th Ed. In: Nordic Committee on Food Analysis, Oslo.
Nordqvist, S. & J. Frishammar (2019). Knowledge types to progress the development of sustainable technologies: a case study of Swedish demonstration plants. International Entrepreneurship and Management Journal, 15:1, pp. 75–95.
Norwegian Food Safety Authority (2020). Matvaretabellen.no, access date 2020-11-18
Nærings- og fiskeridepartementet (2019). “Snøkrabbekvoten øker i 2020”, Regjeringen.no.
Olatunde, O.O., & S. Benjakul (2020). Antioxidants from Crustaceans: A Panacea for Lipid Oxidation in Marine-Based Foods. Food Reviews International, pp. 1-31.
Petrova, I., I. Tolstorebrov & T.M. Eikevik (2018). Production of fish protein hydrolysates step by step: technological aspects, equipment used, major energy costs and methods of their minimizing. International Aquatic Research, 10:3, pp. 223–241.
Rødde, R., A. Einbu & K. Varum (2008). A seasonal study of the chemical composition and chitin quality of shrimp shells obtained from northern shrimp (Pandalus borealis). Carbohydrate Polymers, 71, pp. 388–393.
Sachindra, N.M. & N.S. Mahendrakar (2005). Process optimization for extraction of carotenoids from shrimp waste with vegetable oils. Bioresource Technology, 96:10, pp. 1195–1200.
Semenova, S.A., G.N. Rudenskaya, L.V. Lyutova & O.A. Nikitina (2008). Isolation and properties of collagenolytic serine proteinase isoenzyme from king crab Paralithodes camtschatica. Biochemistry (Moscow), 73:10, pp. 1125–1133.
Shahidi, F. & J. Synowiecki (1991). Isolation and characterization of nutrients and value-added products from snow crab (Chionoecetes opilio) and shrimp (Pandalus borealis) processing discards. Journal of Agricultural and Food Chemistry, 39:8, pp. 1527–1532.
Siikavuopio, S.I., G.S. Johansson, P. James & G. Lorentzen (2019). Effect of starvation on the survival, injury, and weight of adult snow crab, Chionoecetes opilio. Aquaculture Research, 50:2, pp. 550–556.
Souchet, N. & S. Laplante (2011). Recovery and characterization of a serine collagenolytic extract from snow crab (Chionoecetes opilio) by-products. Appl Biochem Biotechnol, 163:6, pp. 765–779.
SSB Norway (2020). “12846: Fangst, etter fangstart og nasjonalitet på fiskefartøyet 2014 – 2019”. In. ssb.no.
Su, W., S. Yu, D. Wu, M. Xia, Z. Wen, Z. Yao, J. Tang & W. Wu (2019). A critical review of cast-off crab shell recycling from the perspective of functional and versatile biomaterials. Environmental Science and Pollution Research, 26:31, pp. 31581–31591.
Svorken, M. & I. E. Pleym (2019). Waste not, want not – an analysis of the marine ingredients industry in Northern Norway. In KBBN – Konjunkturbarometeret for Nord-Norge. https://www.kbnn.no/.
Synowiecki, J. & N.A. Al-Khateeb (2003). Production, Properties, and Some New Applications of Chitin and Its Derivatives. Critical Reviews in Food Science and Nutrition, 43:2, pp. 145–171.
Tremblay, A., R. Corcuff, C. Goulet, S.B. Godefroy, A. Doyen & L. Beaulieu (2020). Valorization of snow crab (Chionoecetes opilio) cooking effluents for food applications. Journal of the Science of Food and Agriculture, 100:1, pp. 384–393.
Vega-Mercado, H., M. Marcela Góngora-Nieto & G.V. Barbosa-Cánovas (2001). Advances in dehydration of foods. Journal of Food Engineering, 49:4, pp. 271–289.
Vilasoa-Martínez, M., J. López-Hernández & M.A. Lage-Yusty (2007). Protein and amino acid contents in the crab, Chionoecetes opilio. Food Chemistry, 103:4, pp. 1330–1336.
Whitaker, R.D., T. Altintzoglou, K. Lian & E.N. Fernandez (2021). Marine Bioactive Peptides in Supplements and Functional Foods – A Commercial Perspective. Current Pharmaceutical Design, 26, pp. 1–12.